Most Life on Earth Is Dormant Right Now
7 min read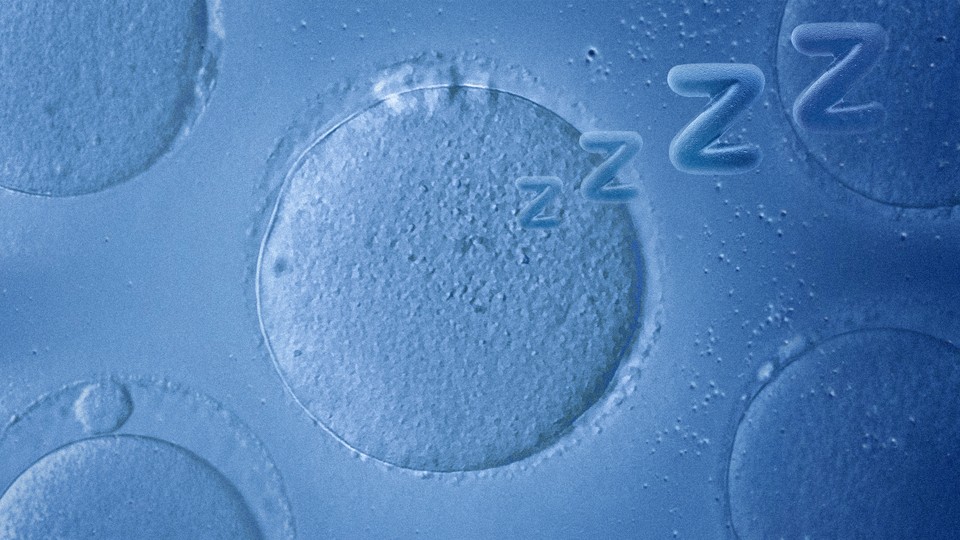
This article was originally published by Quanta Magazine.
Researchers recently reported the discovery of a natural protein, named Balon, that can bring a cell’s production of new proteins to a screeching halt. Balon was found in bacteria that hibernate in Arctic permafrost, but it also seems to be made by many other organisms and may be an overlooked mechanism for dormancy throughout the tree of life.
For most life forms, the ability to shut oneself off is essential to staying alive. Harsh conditions such as lack of food or cold weather can appear out of nowhere. In these dire straits, rather than keel over and die, many organisms have mastered the art of dormancy. They slow down their activity and metabolism. Then, when better times return, they reanimate.
Sitting around in a dormant state is actually the norm for the majority of life on Earth: By some estimates, 60 percent of all microbial cells are hibernating at any given time. Even in organisms whose entire bodies do not go dormant, such as most mammals, some cellular populations within them rest and wait for the best time to activate.
“We live on a dormant planet,” says Sergey Melnikov, an evolutionary molecular biologist at Newcastle University, in the United Kingdom. “Life is mainly about being asleep.”
But how do cells pull off this feat? Over the years, researchers have discovered a number of “hibernation factors,” proteins that cells use to induce and maintain a dormant state. When a cell detects some kind of adverse condition, such as starvation or cold, it produces a suite of hibernation factors to shut down its metabolism.
Some hibernation factors dismantle cellular machinery; others prevent genes from being expressed. The most important ones, however, shut down the ribosome—the cell’s machine for building new proteins. Making proteins accounts for more than 50 percent of energy use in a growing bacterial cell. These hibernation factors throw sand in the gears of the ribosome, preventing it from synthesizing new proteins and thereby saving energy for the needs of basic survival.
The discovery of Balon earlier this year, reported in Nature, presented a new hibernation factor. The protein is shockingly common: A search for its gene sequence uncovered its presence in 20 percent of all cataloged bacterial genomes. And it works in a way that molecular biologists had never seen before.
Previously, all known ribosome-disrupting hibernation factors worked passively: They waited for a ribosome to finish building a protein and then prevented it from starting a new one. Balon, however, pulls the emergency brake. It stuffs itself into every ribosome in the cell, even interrupting active ribosomes in the middle of their work. Before Balon, hibernation factors had been seen only in empty ribosomes.
“The Balon paper is amazingly detailed,” says the evolutionary biologist Jay Lennon, who studies microbial dormancy at Indiana University at Bloomington and was not involved in the new study. “It will add to our view of how dormancy works.”
Melnikov and his graduate student Karla Helena-Bueno discovered Balon in Psychrobacter urativorans, a cold-adapted bacterium native to frozen soils and harvested from Arctic permafrost. (According to Melnikov, the bacterium was first found infecting a pack of frozen sausages in the 1970s and was then rediscovered by the famed genomicist Craig Venter on a trip to the Arctic.) They study P. urativorans and other unusual microbes to characterize the diversity of protein-building tools used across the spectrum of life and to understand how ribosomes can adapt to extreme environments.
Because dormancy can be triggered by a variety of conditions, including starvation and drought, the scientists pursue this research with a practical goal in mind: “We can probably use this knowledge in order to engineer organisms that can tolerate warmer climates,” Melnikov says, “and therefore withstand climate change.”
Helena-Bueno discovered Balon entirely by accident. She was trying to coax P. urativorans to grow happily in the lab. Instead she did the opposite. She left the culture in an ice bucket for too long and managed to cold-shock it. By the time she remembered it was there, the cold-adapted bacteria had gone dormant.
Not wanting to waste the culture, the researchers pursued their original interests anyway. Helena-Bueno extracted the cold-shocked bacteria’s ribosomes and subjected them to cryo-EM. Short for “cryogenic electron microscopy,” cryo-EM is a technique for visualizing minuscule biological structures at high resolution. Helena-Bueno saw a protein jammed into the stalled ribosome’s “A site”—the “door” where amino acids are delivered for the construction of new proteins.
Helena-Bueno and Melnikov didn’t recognize the protein. Indeed, it had never been described before. It bore a similarity to another bacterial protein, one that’s important for disassembling and recycling ribosomal parts, called Pelota from the Spanish for “ball.” So they named the new protein Balon, a different Spanish word for “ball.”
Balon’s ability to halt the ribosome’s activity in its tracks is a crucial adaptation for a microbe under stress, says Mee-Ngan Frances Yap, a microbiologist at Northwestern University who wasn’t involved in the work. “When bacteria are actively growing, they produce lots of ribosomes and RNA,” she says. “When they encounter stress, a species might need to shut down translation” of RNA into new proteins to begin conserving energy for a potentially long hibernation period.
Notably, Balon’s mechanism is a reversible process. Unlike other hibernation factors, it can be inserted to stall growth and then quickly ejected, like a cassette tape. It enables a cell to rapidly go dormant in an emergency and resuscitate itself just as rapidly to readapt to more favorable conditions.
Balon can do this because it latches on to ribosomes in a unique way. Every ribosomal hibernation factor previously discovered physically blocks the ribosome’s A site, so any protein-making process that’s in progress must be completed before the factor can attach to turn off the ribosome. Balon, by contrast, binds near but not across the channel, which allows it to come and go regardless of what the ribosome is doing.
Despite Balon’s mechanistic novelty, it’s an exceedingly common protein. Once it was identified, Helena-Bueno and Melnikov found genetic relatives of Balon in upward of 20 percent of all the bacterial genomes cataloged in public databases. With help from Mariia Rybak, a molecular biologist at the University of Texas Medical Branch, they characterized two of these alternative bacterial proteins: one from the human pathogen Mycobacterium tuberculosis, which causes tuberculosis, and another in Thermus thermophilus, which lives in the last place you’d ever catch P. urativorans—in ultra-hot underwater thermal vents. Both proteins also bind to the ribosome’s A site, suggesting that at least some of these genetic relatives act similarly to Balon in other bacterial species.
Balon is notably absent from Escherichia coli and Staphylococcus aureus, the two most commonly studied bacteria and the most widely used models for cellular dormancy. By focusing on just a few lab organisms, scientists had missed a widespread hibernation tactic, Helena-Bueno says. “I tried to look into an under-studied corner of nature and happened to find something.”
Every cell needs the ability to go dormant and wait for its moment. The laboratory model bacterium E. coli has five separate modes of hibernating, Melnikov says, each of which on its own is sufficient to enable the microbe to survive a crisis.
“Most microbes are starving,” says Ashley Shade, a microbiologist at the University of Lyon, in France, who was not involved in the new study. “They’re existing in a state of want. They’re not doubling. They’re not living their best life.”
But dormancy is also necessary outside periods of starvation. Even in organisms whose entire bodies do not go completely dormant, such as most mammals, individual cellular populations must wait for the best time to activate. Human oocytes lie dormant for decades waiting to be fertilized. Human stem cells are born into the bone marrow and then go quiescent, waiting for the body to call out to them to grow and differentiate. Fibroblasts in nervous tissue, lymphocytes of the immune system, and hepatocytes in the liver all enter dormant, inactive, nondividing phases and reactivate later.
“This is not something that’s unique to bacteria or archaea,” Lennon says. “Every organism in the tree of life has a way of achieving this strategy. They can pause their metabolism.”
Bears hibernate. Herpes viruses lysogenize. Worms develop into a dauer stage. Insects enter diapause. Amphibians aestivate. Birds go into torpor. All of these are words for the exact same thing: a dormant state that organisms can reverse when conditions are favorable.
“Before the invention of hibernation, the only way to live was to keep growing without interruptions,” Melnikov says. “Putting life on pause is a luxury.”
It’s also a type of population-level insurance. Some cells pursue dormancy by detecting environmental changes and responding accordingly. However, many bacteria use a stochastic strategy. “In randomly fluctuating environments, if you don’t go into dormancy sometimes, there’s a chance that the whole population will go extinct” through random encounters with disaster, Lennon says. In even the healthiest, happiest, fastest-growing cultures of E. coli, 5 to 10 percent of the cells will nevertheless be dormant. They are the designated survivors who will live should something happen to their more active, vulnerable cousins.
In that sense, dormancy is a survival strategy for global catastrophes. That’s why Helena-Bueno studies hibernation. She’s interested in which species might remain stable despite climate change, which ones might be able to recover, and which cellular processes—like Balon-assisted hibernation—might help.
More fundamentally, Melnikov and Helena-Bueno hope that the discovery of Balon and its ubiquity will help people reframe what is important in life. We all frequently go dormant, and many of us quite enjoy it. “We spend one-third of our life asleep, but we don’t talk about it at all,” Melnikov says. Instead of complaining about what we’re missing when we’re asleep, maybe we can experience it as a process that connects us to all life on Earth, including microbes slumbering deep in the Arctic permafrost.