How to Cool the World Without Blocking the Sun
6 min read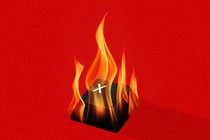
In the fall of 1993, a ship sailed into the Pacific Ocean carrying nearly 1,000 pounds of iron crystals packed into barrels, then dumped it all into the waves. The next morning, the water was tinged a subtle green from newly sprouted phytoplankton. The microorganisms, which need iron to grow, draw carbon dioxide out of the air as they metabolize. Scientists already knew that Earth’s atmosphere was swelling with the gas, and that the planet would soon be in desperate need of cooling down. So why not grow more of the tiny beings that could help? John Martin, the oceanographer who pioneered the idea, put it this way: “Give me a half tanker of iron, and I will give you an ice age.” (He was joking, but not entirely.)
Fertilizing the ocean with iron is a form of geoengineering, a set of technologies that are compelling for their potential to meaningfully alter Earth’s systems, and controversial for the same reason. These days, some geoengineering techniques, such as spraying chemicals into the sky to encourage clouds to produce more rain and ease drought, are already in use. Scientists have begun real-world demonstrations of “cloud brightening,” misting the skies with sea salt to dial up how much sunlight clouds reflect. And even the more controversial approaches—such as injecting the stratosphere with shiny sulfur compounds to block the sun’s rays—are becoming part of mainstream climate discussions. All of these methods are of undetermined efficacy, involve unknown risk, and may entail unintended consequences.
As the planet heats, geoengineering is poised to manipulate it in unprecedented ways, whether researchers invent machines to suck greenhouse gases out of the atmosphere or nudge microorganisms to do it for them. Tinkering with home-grown plankton sounds like a less dramatic approach than, say, putting a massive parasol in outer space to shade the planet from the light of its star. But the field is so new that scientists don’t yet know whether geoengineering with microorganisms would really be a gentler form of climate intervention. Microbes, after all, play enormously consequential roles in the world around us and within us—I should credit the trillions in my body as co-authors of this story. For the best chance of keeping Earth livable, scientists need to understand exactly how the microscopic creatures around us might be useful, and perhaps even preferable to the more sci-fi approaches to cooling off the world.
Microbes, the most abundant organisms on Earth, have been cleaning up human messes since time immemorial, and more recently in ways that help sustain modern life. They decompose the contents of landfills, clean contaminants from bodies of water, break down pollutants in sewage-treatment plants, and eat away at oil spills. In large-scale climate solutions, though, microbes “are horribly underutilized and completely overlooked,” Lisa Stein, a biological-sciences professor at the University of Alberta, told me. Microbes barely factor into the Intergovernmental Panel on Climate Change’s reports on future climate scenarios, Stein said. But she and others say they could have a major role to play in combating human-caused climate change.
One favored approach centers on methane, an extremely potent greenhouse gas that accounts for 30 percent of the rise in global temperature. Mary Lidstrom, a professor emeritus of chemical engineering and microbiology at the University of Washington, is working on genetically modifying bacteria that naturally consume methane as a food source, so that the microbes pull even more gas from the air. The bacteria, known as methanotrophs, would live inside facilities known as bioreactors, which resemble shipping containers. Such bioreactors could be positioned near known methane sources—landfills, coal mines, oil and gas wells, and wetlands—to minimize the amount of methane that makes it into the air. And they could scrub gas that is already circulating in the atmosphere, said Stein, who also serves as Canada’s research chair in climate-change microbiology. The science is still in the early stages, but someday, “it could make a difference in the rate of how fast our thermometer is going up,” she said. Researchers might also exploit carbon-eating microorganisms residing in soils, which hold more carbon than the atmosphere, by modifying soil composition to boost the bacteria’s metabolic capacity.
Bacteria that metabolize carbon could also be of use in the ocean. Matthew Sullivan, a microbiology professor at the Ohio State University, is studying marine viruses that infect such microbes, and influence the way they process carbon. “I’m a fan of leveraging the billions of years of work nature has already done,” Sullivan told me. Perhaps, he said, certain viruses can nudge these bacteria to convert carbon into its heaviest forms. The bacteria would ideally cluster together and sink to the ocean floor, taking the carbon with them so it can’t raise the global temperature.
Scaling up any kind of climate engineering is tricky. Significantly slowing warming caused by methane, for example, would require about 50,000 to 300,000 of Lidstrom’s bioreactors to be active for 20 years. And the downstream effects are difficult to predict, let alone contain. A substance deposited in one plot of land to alter its soil could spread to an entirely different ecosystem, through runoff or some other method, and potentially spoil its happy equilibrium. Similarly, an influx of iron in the South Pacific Ocean could have ramifications for other regions of the world’s seas. Jay Lennon, a biology professor at Indiana University Bloomington, told me that meddling with viruses, too, could have unintended consequences: “Is there going to be a corresponding bloom of viruses that kill off all those phytoplankton and release the carbon dioxide back to the atmosphere?” Such questions are important to consider, but human interventions influence Earth’s microbes all the time, Ruth Varner, a biogeochemistry professor at the University of New Hampshire, told me. For example, every use of fertilizer in agriculture counts as microbial modification. “These are places that we’ve manipulated already,” Varner said.
Much attention is paid to the possibility of geoengineering’s unintended consequences, but there’s nearly as much uncertainty about intended effects. Scientists don’t know for sure how potent many of these concepts will prove to be, including those that have already been tested outside a laboratory. The ship expeditions of the 1990s, for example, didn’t stick around very long; the journeys were expensive, and scientists couldn’t remain at sea to determine what exactly was happening in the depths, David Kirchman, a marine biologist at the University of Delaware, told me. Carbon stolen out of the atmosphere should remain buried for at least a century for maximum impact, he said. Yet it’s entirely possible that if the phytoplankton doesn’t sink to deep enough waters, “it just gets eaten back up by the organisms in the surface ocean, and that CO2 just returns to the atmosphere.”
Scientists haven’t attempted large-scale ocean iron-fertilization experiments since 2012, but the research may soon make a comeback. A pair of researchers are using new funding from the National Oceanic and Atmospheric Administration to model the effectiveness of the technique. There are no sailing trips on the horizon, and yet, microbe research may quickly arrive at the same precipice as other kinds of geoengineering efforts. Real stewardship of Earth’s future lies beyond climate hacks, in making a meaningful shift away from fossil fuels. But the hotter the planet gets, the more attractive geoengineering will likely seem. “Short of large-scale behavioral changes across the planet, we do seem to be committing ourselves to an engineered solution on our current trajectories,” Sullivan said. We might have to decide, sooner than we think, which godlike levers we need to pull, large or small.